GPCR Background Knowledge
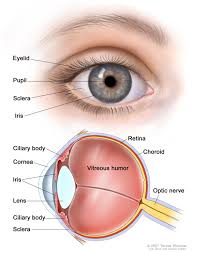
G-protein–coupled receptors (GPCRs) constitute about 50% of the known pharmaceutical drugs and play very important role in a lot of physiological processes like in regulating the heart-beat. Despite its importance in the physiological processes, not much is known about GPCRs since it is challenging to work with GPCRs. Using rhodopsin as a model GPCR, we work on unveiling the mysteries for how the water regulates GPCR activation, how the membrane-lipids- protein interaction influence GPCR functioning, and how the artificial membranes in synthetic systems affect GPCR activation.
New discoveries explain the role of water in rhodopsin activation
Water plays a key role in a lot of biological system like in function of the enzyme, hydrolysis reaction etc. The role of water in rhodopsin activation is not clear. There are contradicting results for the intake or expulsion of water molecules on rhodopsin photoactivation. Osmotic stress studies with small molecular weight osmolytes show that there is efflux of water on rhodopsin activation whereas molecular dynamics studies show there is an influx of water on activation of rhodopsin. For the first time, using different molecular size osmolytes we have shown that influx or efflux of water is size dependent. There is a bulk water intake on activation of the rhodopsin. Hydration-dehydration cycle takes place in the eyes where on activation the inactive Meta-I goes to active Meta-II state with an influx of water. The active Meta-II state has a high affinity for the transducin. Transducin competes with the water just like small osmolytes. Binding of the transducin leads to dehydration of the protein core followed by the exchange of GTP for GDP. The GTP-GDP exchange leads to dissociation of Ga from the dehydrated protein core and leads to inactivated Meta-I state.
Discovering new insights into visual signaling by rhodopsin
Knowing how rhodopsin is activated by light is important for understanding retinal proteins and can contribute added insight into membrane mechanisms. Prior to our work in this area, rhodopsin was regarded to be a simple on-off switch. Using infrared (IR) and electronic (optical) spectroscopic measurements, we found that activation of rhodopsin is not a two-state protein conformational transition. Our team has determined the solid-state NMR structure of the retinal ligand of rhodopsin, and the changes triggered by light activation. Surprisingly, we discovered that an ensemble of conformational states and substrates is affected by the membrane lipid composition, mutations of the protein, and bulk membrane water. To explain our findings, we introduced an ensemble activation model. We have proposed that the retinal cofactor initiates transmembrane helix fluctuations on the microsecond-to-millisecond timescale in visual signaling. Our latest innovations help us to understand how mutations of GPCRs like rhodopsin are implicated in human diseases. Currently, we are applying solid-state NMR methods to investigate how the bound retinal cofactor initiates conformational changes of rhodopsin in its functional mechanism. To acquire a comprehensive picture of rhodopsin signaling in membranes we are combining solid-state NMR, electronic (UV-visible), and Fourier transforms infrared (FTIR) spectroscopy. We are applying our experimental and theoretical techniques to investigate its reactions with light and signaling proteins, including investigations of the role of omega-3 polyunsaturated lipids in visual signaling. By combining spectroscopic methods with functional assays, we aim to achieve an understanding of rhodopsin activation in membranes as a prototype for various membrane proteins.
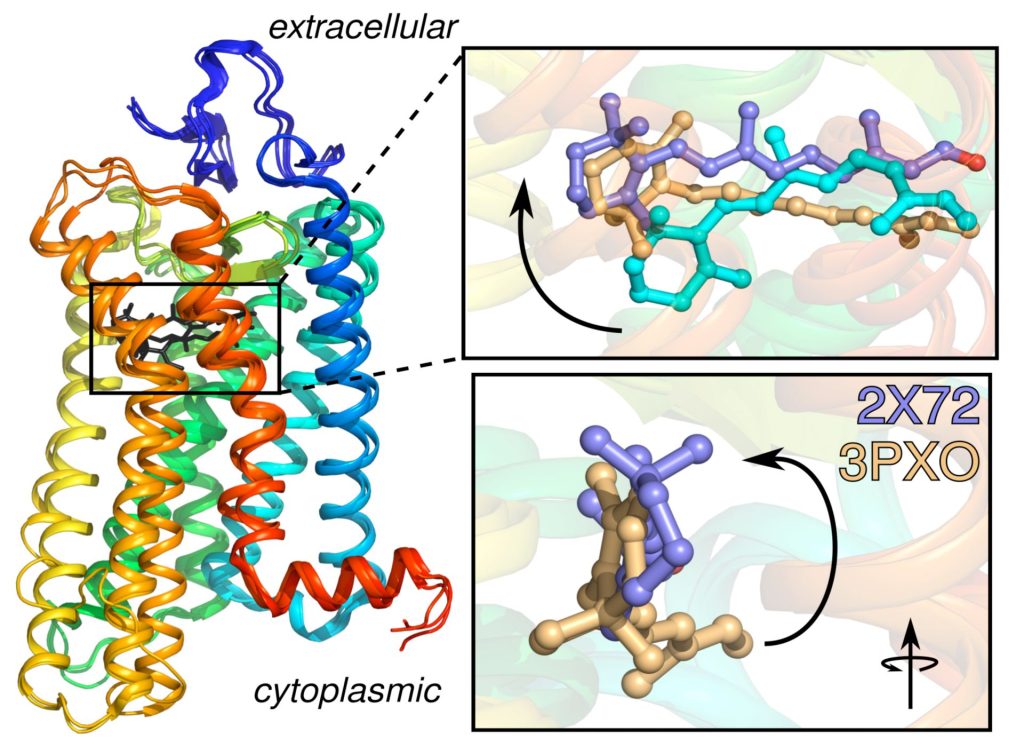
Unraveling the molecular dynamics of G-protein–coupled receptors
Rhodopsin is the prototype for the Family A G-protein–coupled receptors (GPCRs), which are the targets of the majority of human pharmaceuticals. When we began this work, the only X-ray structure for a GPCR was that of rhodopsin in the dark state. Since then, GPCR structures have been appearing at an increasing pace. Understanding the principles of GPCR activation in membranes is significant due to their roles in drug discovery and development. A growing body of evidence supports a key role for dynamics in GPCR function. We have been combining MD simulations with experimental solid-state NMR data to investigate the triggering of visual signaling by rhodopsin. All-atom MD simulations are conducted using supercomputers and graphics processor units (GPUs) to model rhodopsin activation, and its interactions with signaling proteins like transducin. We were first to discover a surprising influx of bulk water into the hydrophobic core of the protein leads to activation of rhodopsin. Currently our research team is conducting molecular dynamics simulations to discover how local motions of the retinal cofactor of rhodopsin yield catalytic activation of transducin. We are testing the relations between hydration, binding affinity, and catalysis of transducin as a basis for understanding visual signaling. Enhanced ligand flexibility upon light activation gives us a ready explanation for the different retinal orientations observed in X-ray crystal structures of active rhodopsin. By combining solid-state NMR studies with molecular dynamics (MD) simulations, we can better understand the receptor activation process.
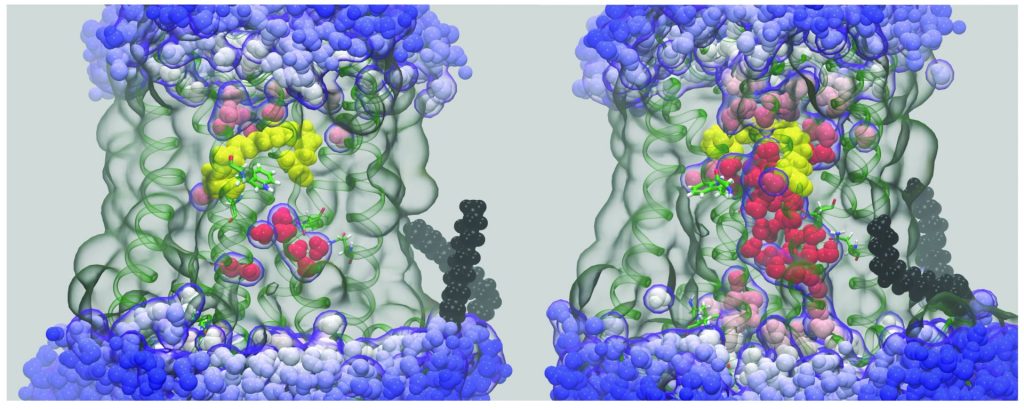